Biomimicry
for shaping future
Agriculture

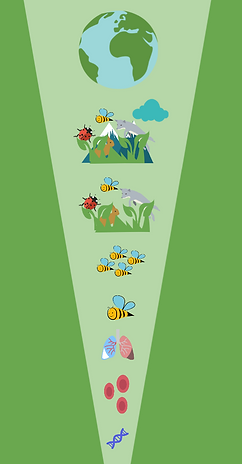
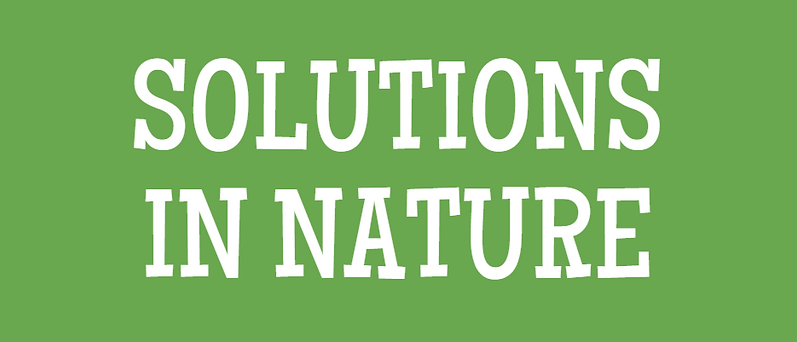
For each of the defined causes of the nutrient cycle problem, we looked for solutions in nature at different levels of organisation:
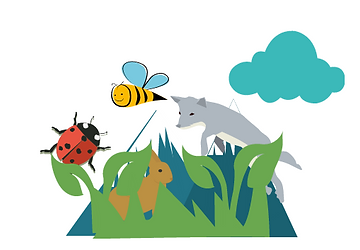
Ecosystem
Input excess
-
Storage in minerals and precipitates.
-
Buffering compounds like carbon in the ocean.
Soil erosion
-
Lower exposure by vegetation cover.
-
Formation of surfaces like desert pavement to prevent further erosion [1]
-
Accelerated soil erosion only occurs when cover levels are altered outside the range of natural variability. [2]
-
Mangroves absorb energy from the waves: a natural breakwater to prevent erosion of the coastline. [3]
-
Retain water in the plot: Building up organic soil matter which holds moisture [4]
-
Agroforestry/polycropping: root systems at different depths hold soil down [4]
Loss of nutrients (by erosion, runoff, harvest, evaporation)
-
Tree and debris dams retain organic carbon within an ecosystem giving coarse particles time to break down [5]
-
Wetlands store carbon faster than terrestrial systems, because of slower decomposition rates under anaerobic conditions and cooler temperatures [6]
-
Efficient irrigation and nutrient application systems: species are adapted to amount of water and nutrients available
Surface water pollution
-
Mangroves extract salt from water via transpiration and filtering through membranes. Also, storage in old leaves that are to be expelled. [7,8]
-
Hedgerows retain nutrients from surface runoff to a great extent [9]
-
Lake ecosystem: A higher input of nutrients causes an increase in algae and a trophic cascade. By adding piscivores (at the top of the cascade) biomanipulation can be carried out to decrease the algae. Zooplankton sinks off to the bottom removing the nutrients out of the system [10, 11].
-
The naturally or artificially vegetated water catchments that retain natural biodiversity in order to obtain pure water are examples of the systems to be mimicked. Tree lots, wind breaks and aquatic vegetation fringing watercourses are all mimics of woodland, swamp, marsh or stream fringing plant communities which may be effective in stripping excess nutrients from drainage lines, streams and waterbodies [12].
Pest control
-
Herbivorous insects in hedgerows in Bavaria help protect nearby wheat fields from aphids by attracting generalist predators that feed on them as well as the aphids [13]
-
Nature reacts to reduced biodiversity or the availability of poorly exploited resources: the system is then open to invasion. Nature sees a monoculture as a species poor environment resulting from an extinction period and tries to increase biodiversity again. It is therefore the question how much biodiversity is enough to prevent invasions, pests and diseases. Manipulation by the farmer will always be necessary. [14,15]
Community
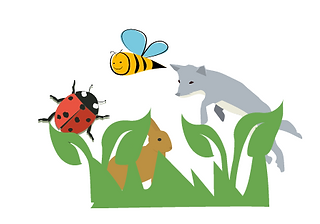
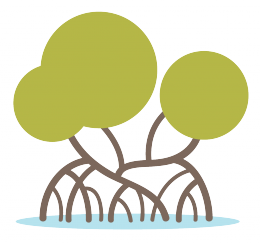
Monocultures/biodiversity
-
Biodiversity in (agro)ecosystems provide many ecosystem services, like nutrient recycling [11]
-
Herbivores change soil microclimate and plant community, resulting in altered nutrient cycling [7]
-
-
Food webs have important effects on nutrient recycling, controlling phytoplankton communities [17]
Fish introduction in lake leads to decreased P limitation of phytoplankton: direct recycling of nutrients and effects on nutrient recycling by zooplankton
Leakage
-
Salamanders are sink of nutrients and are agents in ecosystem nutrient cycling and very efficient in nutrient conversion [4]
Soil erosion and leaching
-
Soil biota (e.g. mycorrhiza) affects soil structure and processes [11]
-
Improve decomposition of organic residue, increase nutrient availability, fix nitrogen, enhance nutrient use efficiency,
entangle particles
-
-
Plant roots and fungi change soil structure and processes [8][14]
-
Influencing microbial activity, release of mycelium products from decomposing hyphae, binding particles, alter water regime
-
-
Fauna change soil properties, e.g. dung beetles, earthworms and termites [9][10][12]
-
Mixing organic residue into the soil, aeration, drainag
-
Excess fertilizer
-
Mycorrhiza fungi (MF) increase plant growth [3][13]
-
Increased decomposition and mineralization of litter
-
Release of nutrients from detrital matter, mainly P for arbuscular MF, mainly N for ecto- and ericoid MF
-

Population
Monoculture
-
Meta-populations theory [6].
-
Separate populations have interactions with nearby populations, increasing survival of the species
-
-
Sensitivity of the distribution and abundance of male cervids to P limitation is predicted to be a function of feeding strategy and body size. [5]
-
Stoichiometric constraints have profound effects on organisms and population
-
Food quality and nutrient recycling feedbacks generated from that, explain population trait variation [2]
-
Stoichimetrically mediated interactions among herbivores can represent mechanism that affect community structure and material/nutrient flows [16]
-
Leaching
-
Soil biota populations can entangle particles, which helps to form aggregates [15].
-
Aggregates are important in nutrient cycling
-
Decomposition in macro-aggregates
-
Stabilisation/sequestration of nutrients (e.g. N and C) in micro-aggregates
-
Excess fertilizer
-
Zooxanthellae (photosynthetic algae) number increases when adding ammonium or ammonium plus phosphate in water [11]
-
That increase is proportional to increase in zooxanthellae proteins. The increased availability of ammonium leads to increased protein synthesis.
-
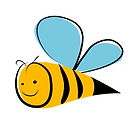
Organism
Leakage
-
Energy in organisms flows via: Ingestion-Egestion = Respiration+Production [4]
-
P limitation is the most severe limitation in ruminants.
-
Low P result in increased P metabolism efficiency in cervids. [5]
-
-
Bamboo clumps are highly efficient in extracting and allocating nutrients from the soil. Nutrients present in leaves are minimal, however, the rhizome system act as a very efficient sink of nutrients [18, 19].
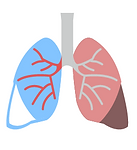
Organ
-
Kidney functioning could be used for
-
Keeping a constant water concentration, by adjusting water removal (drainage).
-
Removing unwanted compounds and reabsorbing necessary compounds [1,2]
-
pH homeostasis. Controlling pH by the excretion of excess acid or base [3].
-
-
pH homeostasis. Adjusting ventilation rate to shift the equilibrium [4,5,6].

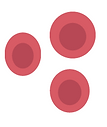
Cell
Leakage
-
Glutamate is present in a high concentration in the brain cells. To prevent leakage by diffusion, a high-affinity uptake system compensates for this [1,2,3,4]
-
Photosynthesis and cellular respiration in a plant cell is a form of carbon cycle within one cell.
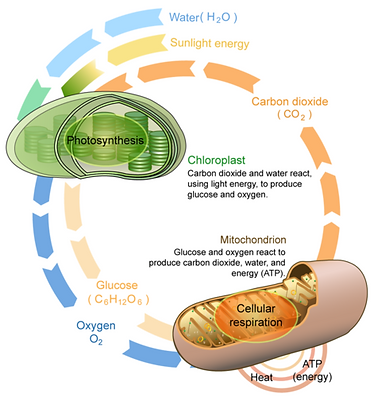
-
Nitrogen storage proteins are present in the bark of trees [5]. These fluctuate seasonally. The storage uncouples nitrogen availability and nitrogen need [6].
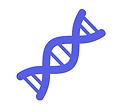
Subcellular
Leakage
-
Neurotransmitter storage capsules are fused with the plasma membrane when the neurotransmitters need to be used. After use active reuptake into cells stores the neurotransmitters back in the storage capsules for later use [1].
-
Circulation of coated pits with receptors. The amount of circulation depends on the availability of resources. The receptors on the membrane of the pits are recycled in the cell membrane [2].
References
Ecosystem
[1] desert pavement. (2015). In Encyclopædia Britannica. Retrieved from http://www.britannica.com/EBchecked/topic/159076/desert-pavement
[2] Webb, N. P., et al. (2014). "Ecological site-based assessments of wind and water erosion: informing accelerated soil erosion management in rangelands." Ecological Applications 24(6): 1405-1420.
[3] Reef Ball Foundation Mangrove Solutions Division. 2007. Why is a mangrove important?. Reef Ball Foundation
[4] Biomimicry Global Design Challenge, 2015. Webinar Food systems.
[5] Bilby, R.E.; Likens, G.E. 1980. Importance of organic debris dams in the structure and function of stream ecosystems. Ecology. 61(5): 1107-1113.
[6] van der Valk, A. 2006. The Biology of Freshwater Wetlands. Oxford: Oxford University Press. 173 p.
[7] Ghosh, D. (2011). "Mangroves." Resonance 16(1): 47-60.
[8] Attenborough, D. 1995. The Private Life of Plants: A Natural History of Plant Behavior. London: BBC Books. 320 p.
[9] Baskin, Y. 1997. The work of nature: how the diversity of life sustains us. Washington, D.C.: Island Press. 263 p
[10] Benndorf, J., Boing, W., Koop, J., and Neubauer, I. 2002. Top-down control of phytoplankton: the role of time scale, lake depth and trophic state. Freshwater Biology 47(2282-2295)
[11] Brett, M.T., and Goldman, C.R. 1996. A meta-analysis of the freshwater trophic cascade. Proc. Natl. Acad. Sci. USA,. 93(7723-7726).
[12] Main, A. R. 1999. How much biodiversity is enough?. Agroforestry Systems. 45(1): 23-41.
[13] Schulze, E-D; Gerstberger, P. 1993. Functional aspects of landscape diversity: A Bavarian example. Pages 453-466 In Biodiversity and Ecosystem Function. In: Schulze, E-D; Mooney, HA, editors. Berlin: Springer-Verlag. p 525
[14] Main, A. R. 1999. How much biodiversity is enough?. Agroforestry Systems. 45(1): 23-41.
[15] MacArthur, RH. 1972. Geographical Ecology. New York: Harper and Rowe.
Community, Population and Organism
[1] Altieri, M. a. (1999). The ecological role of biodiversity in agroecosystems. Agriculture, Ecosystems and Environment, 74(1-3), 19–31. http://doi.org/10.1016/S0167-8809(99)00028-6
[2] Andersen, T., Elser, J. J., & Hessen, D. O. (2004). Stoichiometry and population dynamics. Ecology Letters, 7(9), 884–900. http://doi.org/10.1111/j.1461-0248.2004.00646.x
[3] Bennett, A. E., Alers-Garcia, J., & Bever, J. D. (2006). Three-way interactions among mutualistic mycorrhizal fungi, plants, and plant enemies: hypotheses and synthesis. The American Naturalist, 167(2), 141–152. http://doi.org/10.1086/499379
[4] Burton, T. M., & Likens, G. E. (1975). Energy flow and nutrient cycling in salamander populations in the Hubbard brook experimental forest, New Hampshire. Ecology, 56(5), 1068–1080.
[5] Grasman, B. T., & Hellgren, E. C. (1993). Phosporos nutrition in white-tailed deer: nutrient balance, physiological response, and antler growth. Ecology, 74(8), 2279–2296.
[6] Hanski, I., & Hanski, I. (1998). Metapopulation dynamics. Nature, 396(6706), 41–49. http://doi.org/10.1016/0169-5347(89)90061-X
[7] Hunter, M. D. (2001). Insect population dynamics meets ecosystem ecology: effects of herbivory on soil nutrient dynamics. Agricultural and Forest Entomology, 3(2), 77–84. http://doi.org/10.1046/j.1461-9563.2001.00100.x
[8] Kirkegaard, J., Christen, O., Krupinsky, J., & Layzell, D. (2008). Break crop benefits in temperate wheat production. Field Crops Research, 107(3), 185–195. http://doi.org/10.1016/j.fcr.2008.02.010
[9] Mando, A., & Miedema, R. (1997). Termite-induced change in soil structure after mulching degraded (crusted) soil in the Sahel. Applied Soil Ecology, 6(3), 241–249. http://doi.org/10.1016/S0929-1393(97)00012-7
[10] Milleret, R., Le Bayon, R. C., & Gobat, J. M. (2009). Root, mycorrhiza and earthworm interactions: Their effects on soil structuring processes, plant and soil nutrient concentration and plant biomass. Plant and Soil, 316(1-2), 1–12. http://doi.org/10.1007/s11104-008-9753-7
[11] Muscatine, L., Falkowski, P. G., Dubinsky, Z., Cook, P. A., & McCloskey, L. R. (1989). Muscatine et al 1989- Effect of external nutrient resources on the population dynamicx of zooxanthellae. Proceedings of the Royal Society Biological Sciences, 236, 311–324.
[12] Nichols, E., Spector, S., Louzada, J., Larsen, T., Amezquita, S., & Favila, M. E. (2008). Ecological functions and ecosystem services provided by Scarabaeinae dung beetles. Biological Conservation, 141(6), 1461–1474. http://doi.org/10.1016/j.biocon.2008.04.011
[13] Read, D. J., & Perez-Moreno, J. (2003). Mycorrhizas and nutrient cycling in ecosystems - A journey towards relevance? New Phytologist, 157(3), 475–492. http://doi.org/10.1046/j.1469-8137.2003.00704.x
[14] Rillig, M. C., & Mummey, D. L. (2006). Tansley review Mycorrhizas and soil structure. New Phytologist, 41–53.
[15] Six, J., Elliott, E. T., & Paustian, K. (2000). Soil macroaggregate turnover and microaggregate formation: A mechanism for C sequestration under no-tillage agriculture. Soil Biology and Biochemistry, 32(14), 2099–2103. http://doi.org/10.1016/S0038-0717(00)00179-6
[16] Urabe, J., Elser, J. J., Kyle, M., Yoshida, T., Sekino, T., & Kawabata, Z. (2002). Herbivorous animals can mitigate unfavourable ratios of energy and material supplies by enhancing nutrient recycling. Ecology Letters, 5(2), 177–185. http://doi.org/10.1046/j.1461-0248.2002.00303.x
[17] Vanni, M. J., & Layne, C. D. (1997). Nutrient recycling and herbivory as mechanisms in the “top-down” effect of fish on algae in lakes. Ecology, 78(1), 21–40. http://doi.org/10.1890/0012-9658(1997)078[0021:NRAHAM]2.0.CO;2
[18] Tripathi S. and Singh K. (1994) Productivity and Nutrient Cycling in Recently Harvested and Mature Bamboo Savannas in the Dry Tropics. The journal of Applied Ecology, 31(1) p.109.
[19] Shanmughavel P. (2001) Bioproductivity and nutrient cycling in bamboo and acacia plantation forests. Bioresource Technology, 80(1), p.45-48.
Organ
[1] Berliner, R. W., Levinsky, N. G., Davidson, D. G., & Eden, M. (1958). Dilution and concentration of the urine and the action of antidiuretic hormone. The American journal of medicine, 24(5), 730-744.
[2] Shannon, J. A., & Fisher, S. (1938). The renal tubular reabsorption of glucose in the normal dog. American Journal of Physiology--Legacy Content, 122(3), 765-774.
[3] Soriano, J. R. (2002). Renal tubular acidosis: the clinical entity. Journal of the American Society of Nephrology, 13(8), 2160-2170.
[4] Idris, A. H., Staples, E. D., O'brien, D. J., Melker, R. J., Rush, W. J., Del Duca, K. D., & Falk, J. L. (1994). Effect of ventilation on acid-base balance and oxygenation in low blood-flow states. Critical care medicine, 22(11), 1827-1834.
[5] Leusen, I. R. (1953). Chemosensitivity of the respiratory center. American Journal of Physiology--Legacy Content, 176(1), 45-51.
[6] Wood, C. E., & Chen, H. G. (1989). Acidemia stimulates ACTH, vasopressin, and heart rate responses in fetal sheep. American Journal of Physiology-Regulatory, Integrative and Comparative Physiology, 257(2), R344-R349.
Cell
[1] Bradford, H. F., Young, A. M. J., & Crowder, J. M. (1987). Continuous glutamate leakage from brain cells is balanced by compensatory high-affinity reuptake transport. Neuroscience letters, 81(3), 296-302.
[2]Danbolt, N. C. (1994). The high affinity uptake system for excitatory amino acids in the brain. Progress in neurobiology, 44(4), 377-396.
[3]Logan, W. J., & Snyder, S. H. (1971). Unique high affinity uptake systems for glycine, glutamic and aspartic acids in central nervous tissue of the rat. Nature,234, 297-299.
[4] Wofsey, A. R., Kuhar, M. J., & Snyder, S. H. (1971). A unique synaptosomal fraction, which accumulates glutamic and aspartic acids, in brain tissue.Proceedings of the National Academy of Sciences, 68(6), 1102-1106.
[5] Wetzel, S., Demmers, C., & Greenwood, J. S. (1989). Seasonally fluctuating bark proteins are a potential form of nitrogen storage in three temperate hardwoods. Planta, 178(3), 275-281.
[6]Millard, P., & Grelet, G. A. (2010). Nitrogen storage and remobilization by trees: ecophysiological relevance in a changing world. Tree Physiology, 30(9), 1083-1095.
Subcellular
[1] Kanner, B. I., & Schuldiner, S. (1987). Mechanism of transport and storage of neurotransmitter. Critical Reviews in Biochemistry and Molecular Biology,22(1), 1-38.
[2] Brown, M. S., Anderson, R. G., & Goldstein, J. L. (1983). Recycling receptors: the round-trip itinerary of migrant membrane proteins. Cell, 32(3), 663-667.


