Biomimicry
for shaping future
Agriculture
Biomimicry Global Design Challenge
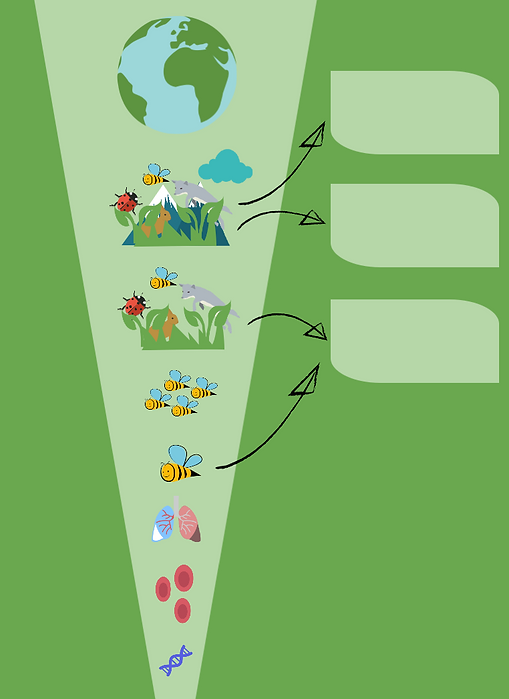
We developped a to better evaluate the potential of each idea.
Three emerging ideas at different level of organisation:
1. Inspiration from spatial patterns in nature
Living beings have developed a large diversity of designs, patterns and colours. In biology, such regularities can serve for camouflage, sexual selection, pollination, or various kind of signalling (Darwin, 1859) but patterns also occur at a much larger scale, such as at landscape level.
The term “landscape mosaic” describes the pattern of patches, matrices, corridors, and stepping stones associated with the underlying landform; it is particularly interesting since these environmental distributions largely determines ecological functions and processes and greatly influence the flow of resources and species (Turner, 1989; Bell & Apostol, 2008). Organisms live in spatially structured habitats (at different scales), and these arrangements interact with the perception and behaviour of those organisms such as it drives population dynamics and community structure (Johnson et al. 1992). Some patterns repeat itself at different scales, highlighting the fact that some particular shapes have a clear advantage. Human activities have sometimes disrupted the integrity of these landscape structures, thereby compromising their functioning by interfering with critical ecological processes necessary for population persistence and the maintenance of biodiversity and ecosystem function (With, 1997).
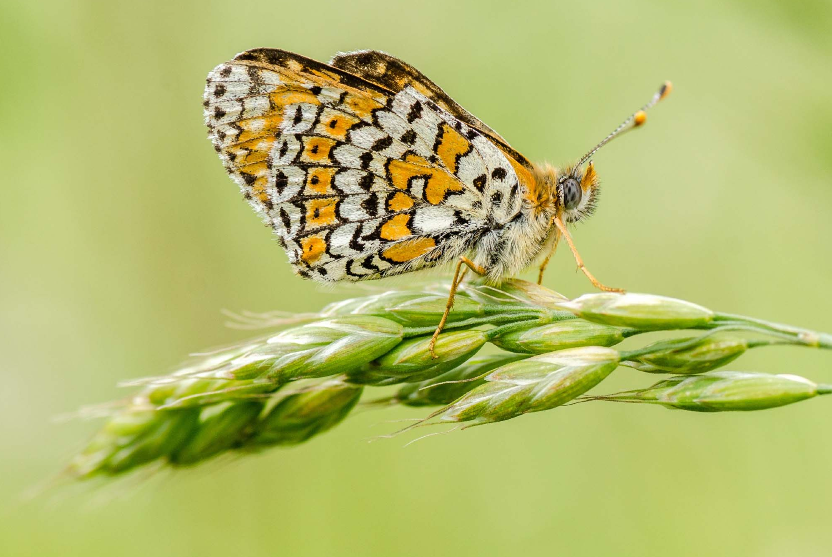
Ecological Methods 1, WageningenUR
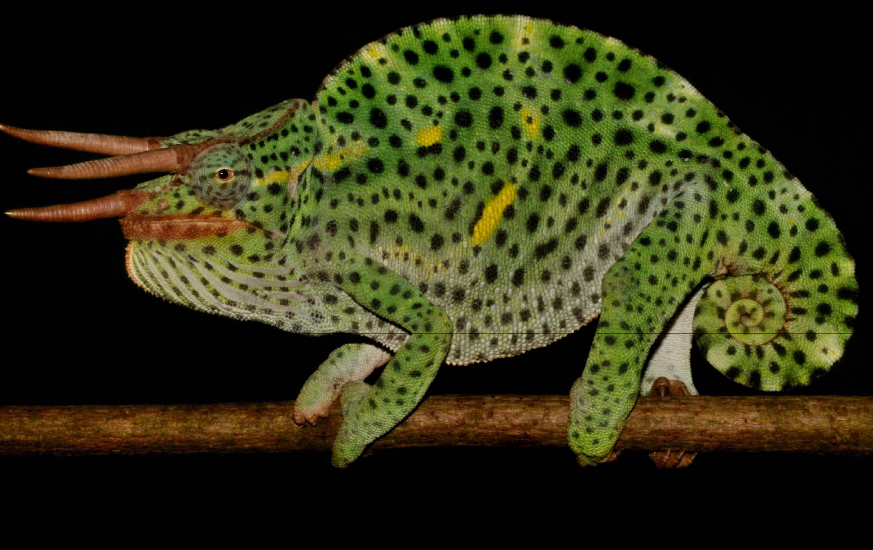
Ecological Methods 1, WageningenUR
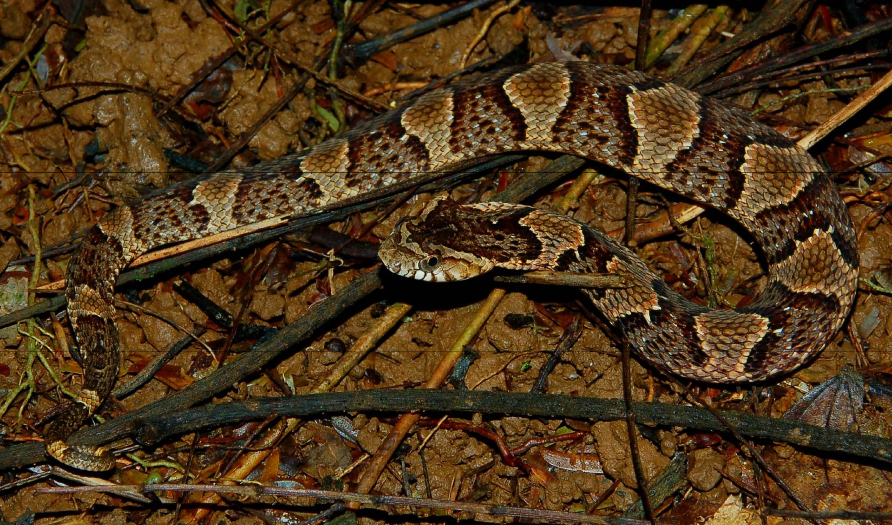
Ecological Methods 1, WageningenUR
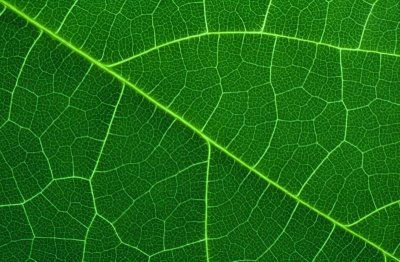
http://www.pics4world.com

Ecological Methods 1, WageningenUR
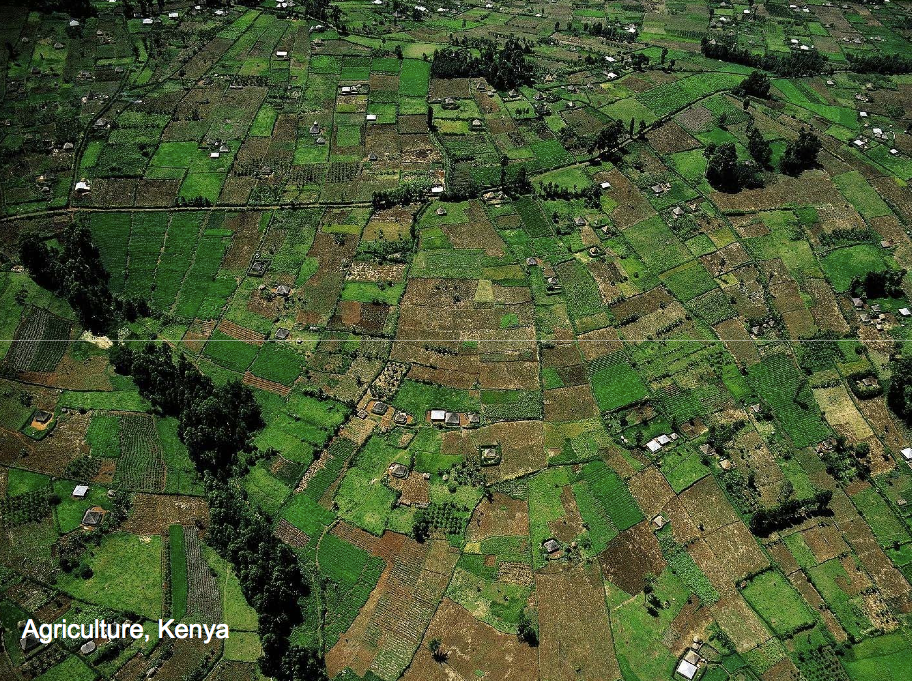
Ecological Methods 1, WageningenUR
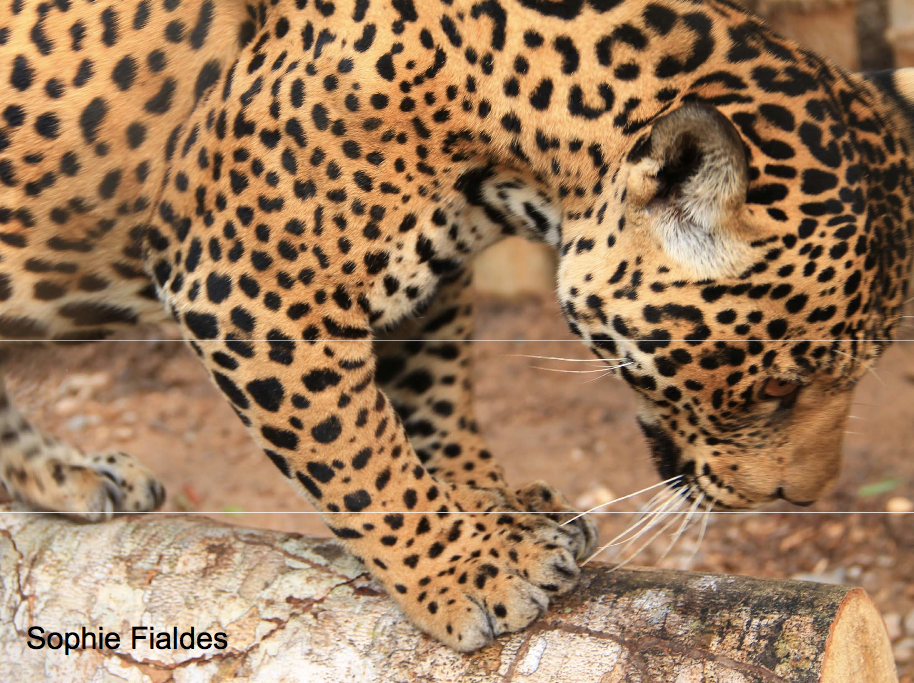
Ecological Methods 1, WageningenUR
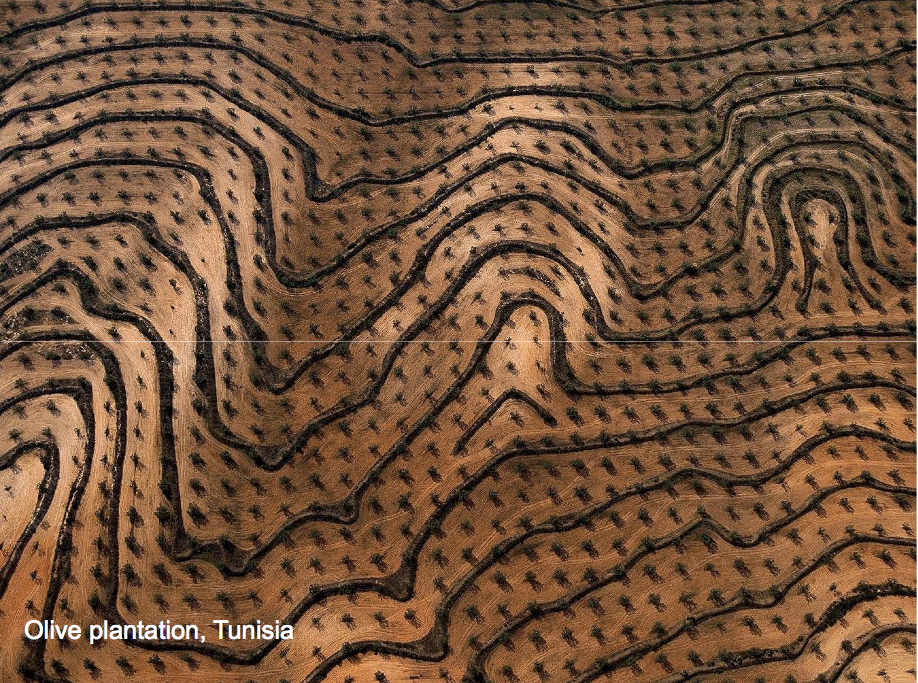
Ecological Methods 1, WageningenUR
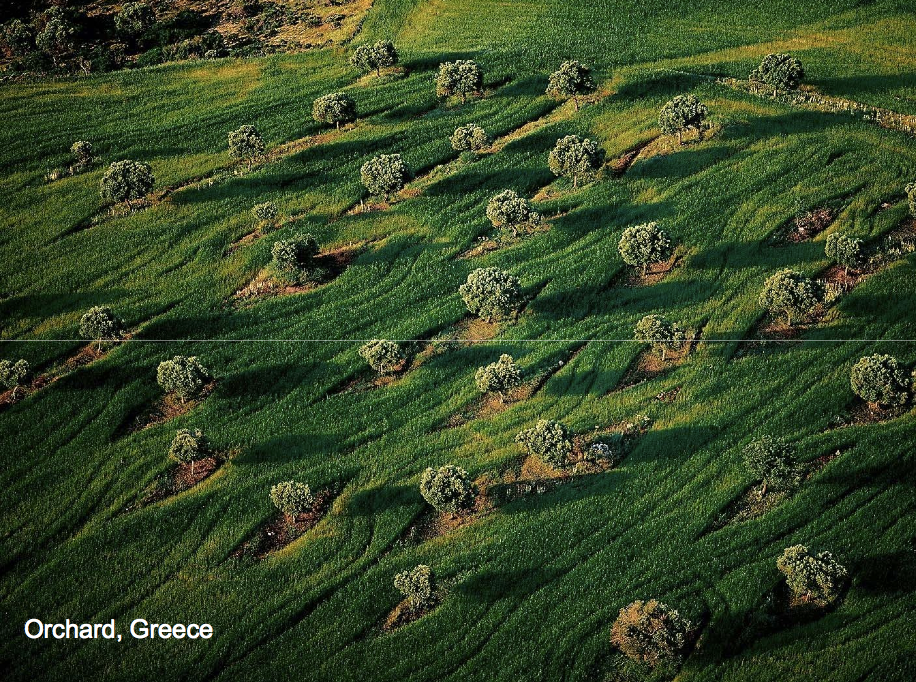
Ecological Methods 1, WageningenUR
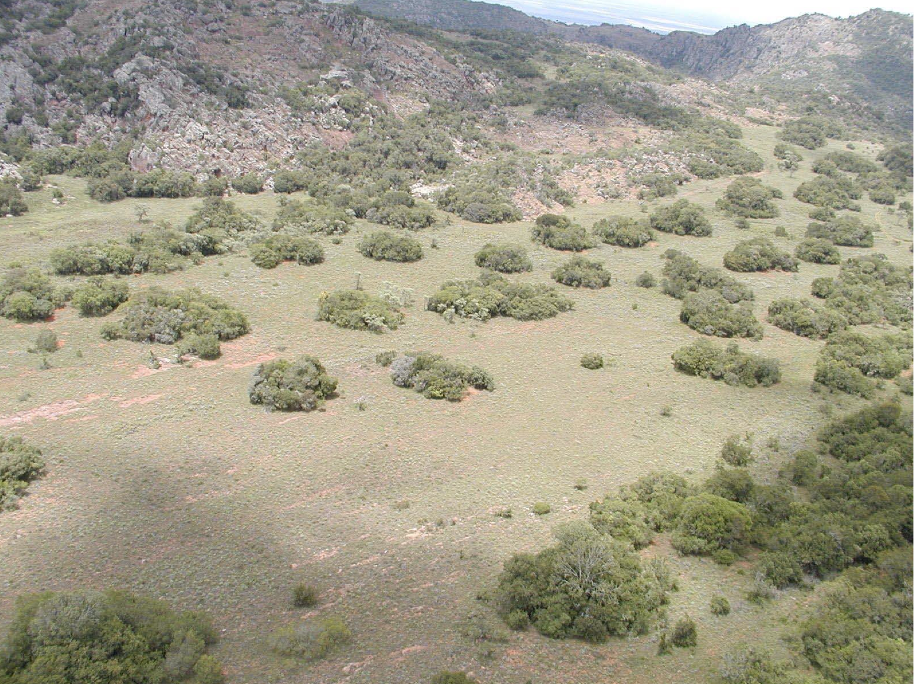
Ecological Methods 1, WageningenUR

Ecological Methods 1, WageningenUR

http://www.pics4world.com
The intensification of the agricultural sector has led to a simplification of the landscape by expanding agricultural lands, enlarging the size of the fields, and suppressing non-crop habitats (Bianchi et al., 2006). As a result, farmlands have been subject to a decline in biodiversity and a reduction of natural pest control. Indeed, a broad spectrum of natural enemies is concentrated in non-crop habitats, and with a reduction of contact between crop and non-crop habitats due to landscape simplification the exchange of natural enemies between those two systems reduced. Bianchi et al. (2006) show that natural enemy populations are higher and pest pressure lower in complex landscapes versus simple landscapes. They also showed that herbaceous habitats (e.g. fallows, field margins), wood habitats, and landscape patchiness are important factors in enhancing natural enemy populations.
Therefore by analysing patterns that occur in nature we will be able to find the most suitable landscape pattern that meets both agricultural and nature conservation requirements in order to conserve or increase biodiversity, develop a sustainable pest control and optimize the agricultural area.
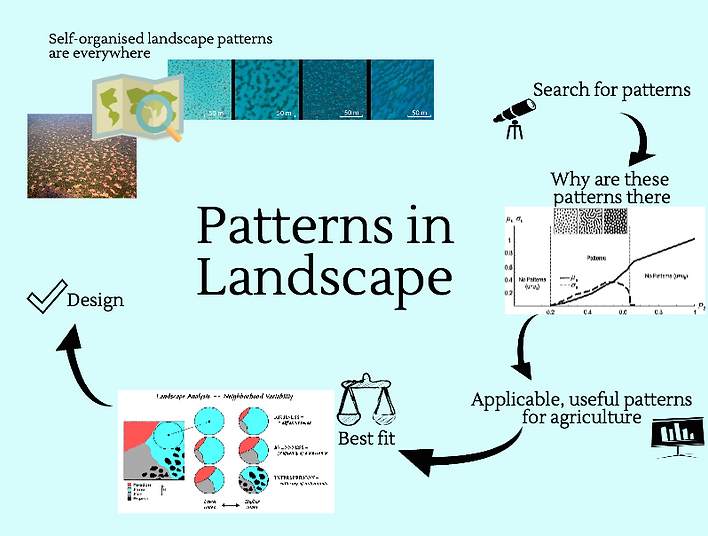
2. Nature-like closed-cycles with algae/nutrients cycle
However, current agricultural practices deplete the soil of its nutrients through the harvest of the crops, erosion of the soils, runoff at the surface, and leaching inside the soil; making agriculture unsustainable over the long term (Gruhn et al., 2000; Pedro et al., 1997). According to Gitelson & Lisovsky (2003), the key solution for sustainably developing civilization on our planet is through the principle of balanced material cycle.
The Earth's resources are limited.
Life on Earth, on the other hand, seems endless.
This paradox is made feasible only because the resources accessible to life can be used again and again through material cycles (Gitelson & Lisovsky, 2003).
Water derived from the land surface and ground is enriched with soil particles, which are necessary for the soil quality and crop production (Tan et al., 2005). This water often runs off from farms and fields ending up in neighbouring ditches and streams, particularly during raining periods (Pimentel, 2006). Water leaching causes nutrient depletion in the agricultural soils and consequently, reduces the crop yield (Tan et al., 2005). The idea of using algae in order to prevent the nutrient losses from the soils is based on the fact that they are photosynthetic, so using them to convert the minerals that are removed from the agricultural field into biomass that can be used as organic fertiliser seems to be a promising solution to the nutrient loss. This means a more efficient cycling of nutrients in agricultural systems.
Another aspect of introducing algae in agriculture aims to “hijack” algae ability in using compounds in the water for their growth and reproduction (Bold et al., 1985). In this way, the agricultural wastewater is cleaned up from the agrochemical fertilizers and residues from manure, leading to the suppression of the detrimental effect of agriculture in the environment.
Implementation of this technology would be done by using tank-filters that contain algae cultures in controlled conditions. Agricultural wastewater passes through, carrying the leached nutrients which are incorporated by these organisms. Algae is harvested and processed into fertilizer which is used on the same agricultural fields, while the remaining water is clean enough to prevent eutrophication in water bodies. Dependency on external inputs is diminished (although not completely eliminated) as the nutrient cycle is more enclosed in the agricultural system, while the risks of pollution of the local watershed are diminished.

3. Beehive inspiration for hexagonal patterns

![]() Tree Flowers | ![]() Autumn Leaves |
---|---|
![]() Fabaceae | ![]() Tranquil forest |
![]() honeycomb | ![]() Echinacea Coneflowers |
![]() Fresh Herbs Close Up | ![]() nature |
![]() Lush Plants | ![]() Open Field |
![]() Berries close Up | ![]() Stalks of Wheat |
For our second design, we got inspired by the structure of honeycomb to create a polyculture field with hexagonal patches. The rationale behind this specific pattern relies on three principles: the so-called honeycomb conjecture, a regular partitioning of the land and the maximal use of space with high number of interactions.
So creating a complex landscape using this design would bring lots of benefits in terms of biodiversity, biological pest control, and complex trophic networks leading to the increase of the resilience of the system.
Nevertheless further analysis is needed to determine an optimal hexagon size to find the best way to fit practical issues like harvesting and infrastructures needed, to ensure the interaction between each plot and how to establish this pattern on the land.
The first principle, which is an explanation for the geometry of the the actual honeycombs, reveals that an hexagonal grid is the optimal way for dividing a surface into regions of the same area with the least total perimeter (Mathworld.wolfram.com, 2015). This complements the second reasoning in which the land can be divided into regular sections, practical for farming activities. Finally, by this way all the land can be used and managed while the interactions between each plot are increased.
Interactions within ecosystems are crucial; enhancing and increasing them is of primary importance in agrosystems that strongly relies on internal processes. With this particular structure, each hexagon is bordered by six other plots, each one receiving different ecoservices. It also allows the rotation of 6 different crops around one central perennial plot, or would be the base of a stable community with 7 perennial productive species.


References
Bell S. & Apostol D. (2008) Designing sustainable forest landscapes.
Bianchi FJ, Booij CJ, Tscharntke T. (2006) Sustainable pest regulation in agricultural landscapes: a review on landscape composition, biodiversity and natural pest control. Proc Biol Sci. 273(1595): 1715-27.
Bold H. C., & Wynne M. J. (1985). Introduction to the algae: structure and reproduction. Prentice-Hall.
Darwin C. (1859) On the origin of species by means of natural selection, or the preservation of favoured races in the struggle for life. London: Murray.
Gaucherel C., Boudon F., Houet T., Castets M., Godin C. (2012) Understanding Patchy Landscape Dynamics: Towards a Landscape Language. PLOS One DOI: 10.1371/journal.pone.0046064.
Gitelson J. and Lisovsky G. (2003) Man-Made Closed Ecological Systems. Hoboken: Taylor and Francis.
Gruhn P., Goletti F., and Yudelman M. (2000) Integrated Nutrient Management, Soil Fertility, and Sustainable Agriculture: Current Issues and Future Challenges. Food, Agriculture, and the Environment Discussion Paper 32, International Food Policy Research Institute, Washington, D.C.
Johnson A.R., Milne B.T., Wiens J.A., Crist T.O. (1992) Animal movements and population dynamics in heterogeneous landscapes. Landscape Ecology, 7: 63-75.
Mathworld.wolfram.com (2015). Honeycomb Conjecture -- from Wolfram MathWorld. [online] Available at: http://mathworld.wolfram.com/HoneycombConjecture.html [Accessed 10 Apr. 2015].
Pedro et al. (1997) Trees, soils and food security. Biological Sciences 352 No.1356: 949-961.
Pimentel D. (2006). Soil erosion: a food and environmental threat. Environment, development and sustainability, 8(1), 119-137.
Tan Z.X., Lal R., & Wiebe K.D. (2005). Global soil nutrient depletion and yield reduction. Journal of Sustainable Agriculture, 26(1), 123-146.
Turner M.G., (1989) Landscape ecology: the effect of pattern on process. Ann. Rev. Ecol. Syst. 20, 171–197.
With K.A. (1997) The application of neutral landscape models in conservation biol- ogy. Conserv. Biol. 11, 1069–1080.


